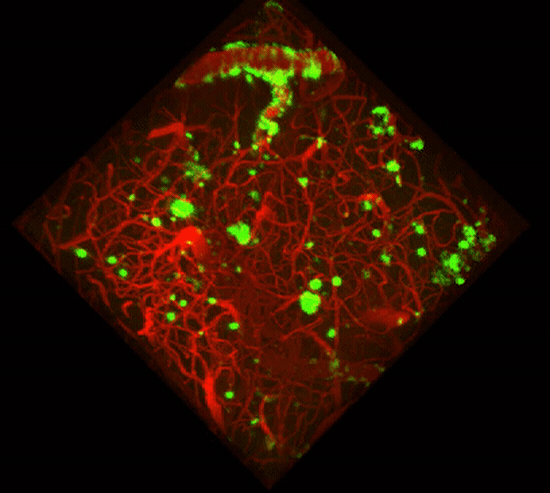
Two-photon imaging of Aβ deposits in the brain of a living mouse. Data obtained at Neurotar.
We use in vivo two-photon microscopy to visualize the three-dimensional distribution of fluorescently labeled Amyloid-beta (Aβ) plaques (also known as Abeta plaques). We can track changes in the plaque size and number over weeks to months, and quantify the plaques’ co-localization with fluorescently labeled molecules such as anti-Abeta antibodies. Besides that: it is also possible to measure plaques’ proximity to anatomical structures such as cortical neurons or blood vessels. This assay is particularly useful for preclinical studies of biodistribution and target engagement, MoA, and Abeta plaques’ pharmacodynamics.
Amyloid-beta and tau targeting strategies in AD research
Amyloid-beta (Aβ) and tau proteins are key hallmarks of Alzheimer’s disease (AD) and targets of multiple disease-modifying therapies. Recent breakthroughs with Abeta-targeting therapeutics like Aducanumab, Donanemab, and Lecanemab have showcased their potential to slow disease progression. They offered renewed hope for effective treatments.
Both Abeta- and tau-targeting strategies rest on the premise that managing the accumulation or promoting the clearance of Aβ and tau in the brain could improve cognitive function and slow disease progression. Critical to these approaches are advanced imaging techniques such as MRI, PET, and multiphoton imaging, also known as two-photon microscopy. Imaging approaches stand out for their ability to quantitatively measure Aβ plaque and tau tangle burdens over time. They offer these readouts within the physiologically relevant context of the living brain.
Why study the Abeta plaques with two-photon microscopy?
Conventional imaging methods such as Positron-Emission Tomography (PET) can measure the overall Abeta plaque load in vivo (Ni, 2021). However, only two-photon microscopy offers micrometer-scale spatial resolution. High resolution enables mechanistic in vivo studies of individual plaques. These studies can follow plaques’ formation, growth, and proximity to blood vessels or other fluorescently labeled structures, cells, and molecules. Importantly, two-photon microscopy is the only imaging technique to achieve a clear spatial distinction between perivascular versus parenchymal Abeta plaques. These different plaque types are known to have distinct contributions to the overall plaque load and AD pathogenesis (Thal et al, 2008). When it comes to learning such crucial details about the interaction of your Abeta-targeting biologics or small-molecule drugs with Abeta plaques, there is simply no alternative to the in vivo two-photon microscopy.
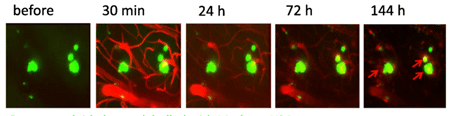
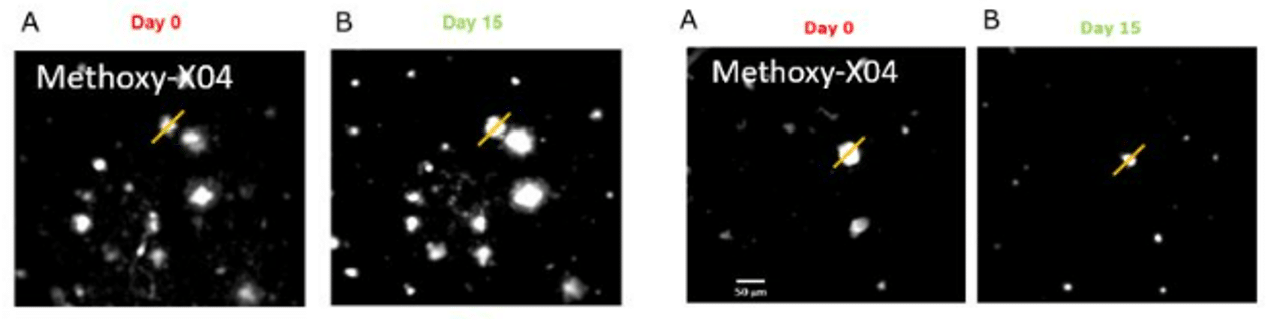
Targeting Tau instead of Abeta? Follow this link to learn about our tau dynamics imaging assay.
What types of studies can Neurotar perform for Abeta-targeting drug developers?
The study design starts with an assessment of your molecule’s size. It is possible to label the relatively large biological molecules with a fluorescent tag to add an extra pharmacokinetic/biodistribution dimension to a study. Measuring plaque proximity to anatomical structures such as cortical neurons or blood vessels requires additional labeling. It may be vessel labeling with fluorescently labeled dextrans for the latter or neuronal labeling (such as a GFP) for the former.
The most popular options for our clients’ Abeta imaging studies include:
A popular extension for the Abeta imaging studies is the addition of behavioral observations such as hind paw clasping or weight loss.
Additionally, our assays are highly suitable for detailed in vivo characterization of novel transgenic mouse models of AD. Our recent in vivo validation of reMYND‘s novel humanized App knock-in mouse model (AppSAA) is a good illustration of this approach. This novel AppSAA model endogenously expresses APP with three clinical mutations.
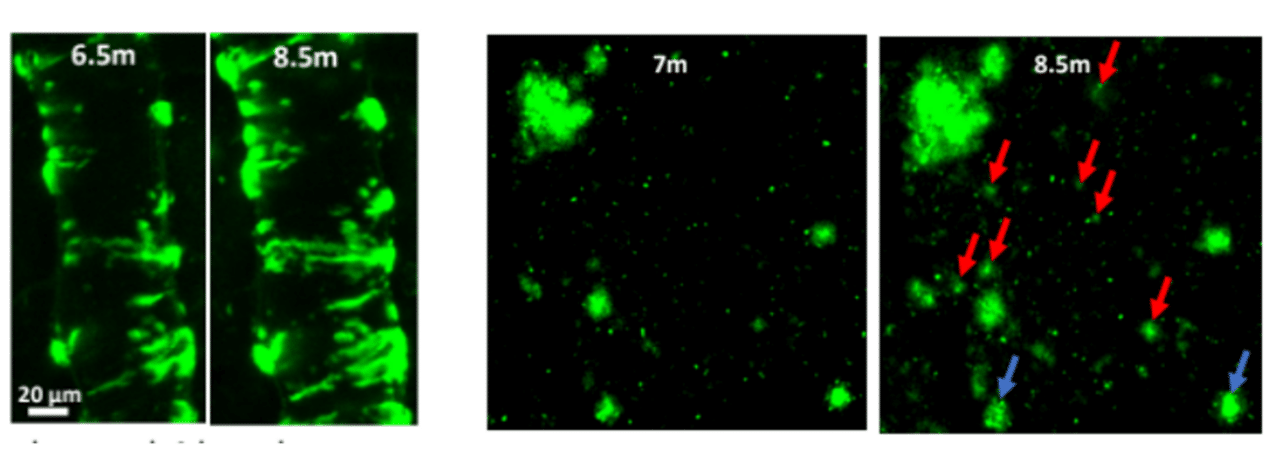
Interested to learn more about this model and the recent validation project? – Meet reMYND and Neurotar teams at the AD/PD Conference in Lisbon, Portugal from March 5-9, 2024. Won’t make it to AD/PD conference? – Contact us for a poster abstract.
How can Neurotar facilitate your in vivo studies on Abeta plaque-expressing mouse models?
First, we’ll sit down with you for a thorough discussion of your research questions. Together, we will formulate the hypothesis and design an optimally powered in vivo microscopy study for testing your hypothesis.
We may then ask you to provide a fluorescently labeled version of your biomolecule, while we prepare the transgenic mice by implanting them with cranial windows.
Unless you already have access to your preferred AD mouse line, we will help you source the mice from a validated provider of AD mouse models such as Tg2576, 5xFAD, or APPSL.
Finally, upon completion of the study, we shall present the results and propose our interpretation of the data. We shall then answer all of your questions and finalize the report based on your comments and requests. If you’d like to publish some or all of the study results, we’ll be delighted to assist you in writing the Materials and Methods section.
Publications by Neurotar:
Pradier, L., Blanchard-Brégeon, V., Bohme, A. et al. (2018) SAR228810: an antibody for protofibrillar amyloid β peptide designed to reduce the risk of amyloid-related imaging abnormalities (ARIA). Alz Res Therapy 10, 117. https://doi.org/10.1186/s13195-018-0447-y
Gureviciene I, Gurevicius K, Mugantseva E, Kislin M, Khiroug L, Tanila H. (2017) Amyloid Plaques Show Binding Capacity of Exogenous Injected Amyloid-beta. J Alzheimers Dis. 55(1):147-157. https://doi.org/10.3233/JAD-160453.
Pryazhnikov E, Julku U, Kopcsanyi T, Cornelissen T, Dejonckheere W, Carmans S. (2024) Longitudinal in vivo microscopic imaging of Aβ pathology in a novel humanized App knock-in mouse model (AppSAA). Poster presentation #2793. AD/PD 2024, March 5-9, Lisbon, Portugal. AD/PD Conference
Other relevant publications:
Ni K. (2021) Positron Emission Tomography in Animal Models of Alzheimer’s Disease Amyloidosis: Translational Implications. Pharmaceuticals (Basel) 14(11):1179. https://doi.org/10.3390/ph14111179
Thal D.R., Griffin W.S.T., Braak H. (2008) Parenchymal and vascular Aβ-deposition and its effects on the degeneration of neurons and cognition in Alzheimer’s disease. J Cell Mol Med. 12(5b): 1848–1862. https://doi.org/10.1111/j.1582-4934.2008.00411.x
Other two-photon brain imaging services offered by Neurotar
Neurodeg. Disease (e.g. AD, PD) | Stroke and TBI | Neuropathic Pain and Migraine | Neuropsychiatry (e.g. Schizophrenia) | Epilepsy | |
---|---|---|---|---|---|
Blood-brain barrier integrity | ✓ | ✓ | ✓ | ||
Trans-BBB pharmacokinetics | ✓ | ✓ | ✓ | ||
Dendritic spine turnover | ✓ | ✓ | ✓ | ✓ | ✓ |
Microglial dynamics or response to injury | ✓ | ✓ | ✓ | ✓ | ✓ |
Calcium signaling | ✓ | ✓ | ✓ | ✓ | ✓ |
Abeta Plaque or Tau Tangle dynamics | ✓ | ✓ | ✓ | ||
Mitochondrial fragmentation | ✓ | ✓ | ✓ | ✓ | ✓ |
Ischemic Stroke model | ✓ | ||||
Regeneration of peripheral neurons | ✓ | ✓ | ✓ |